Research HighlightsGenome Editing
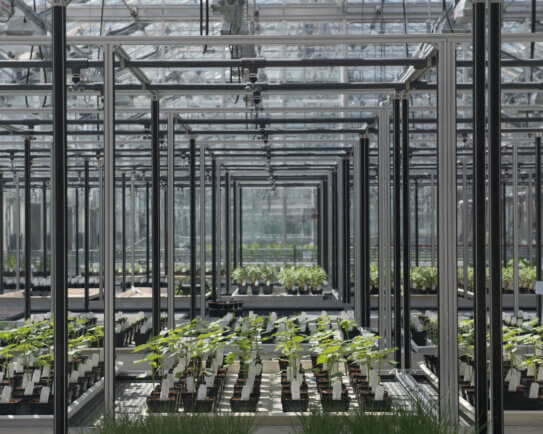
By Ed Anderson, Iowa Soybean Association Senior Director of Research
Humans have manipulated and modified the genetic material of plants and animals for thousands of years to improve yield, health, quality and overall performance. From simple selections to classical breeding to genetic engineering and biotechnology, scientists have constantly strived to increase the speed, precision, accuracy and efficiency of plant and animal improvement.
A new set of biotechnology tools has been classified “genome editing” because they enable researchers to make precise modifications in an organism’s DNA relatively quickly and easily. Genome edits include small genetic insertions and deletions, as well as small or large gene modifications and replacements. The most common techniques in this new class of genome editing tools include: meganucleases, zinc finger nucleases (ZFNs), transcription activator-like effector-based nucleases (TALENs) and clustered regularly interspaced short palindromic repeats (CRISPR/Cas9). All of these rely on special “molecular scissors” called nucleases that cut one or both strands of a double-stranded DNA molecule at predetermined locations and allow subsequent modifications. Since the Clustered Regularly Interspaced Short Palindromic Repeats (CRISPR) technology has emerged as the most powerful and versatile of these, we will focus on it in this article.
CRISPR in nature
Like many biotechnology tools, CRISPR was invented by nature and then discovered and repurposed by scientists. In nature, bacteria use CRISPR-associated nucleic acid and protein as part of a rather elegant defense system against infecting viruses by recognizing and destroying an infecting virus’ genetic material. There are two key features of this defense process. First, the CRISPR-related bacterial nucleic acid (in this case, RNA) specifically recognizes and attaches to certain virus RNA or DNA sequences. Second, when the CRISPR RNA attaches to the invading virus’ nucleic acid, it ‘guides’ a CRISPR-related protein nuclease (Cas9 or similar enzyme) that cuts the virus DNA or RNA to block the infection.
CRISPR in biotechnology
In biotechnology, scientists learned quite some time ago that naturally occurring gene modifying systems can be manipulated for our benefit. Once scientists studied and learned that the fundamental mechanisms of CRISPR involve nucleic acid sequence-dependent, site-specific recognition and cutting, they purified, characterized and customized the CRISPR components to modify plant and animal genomes in precise fashion (Figure 1). Thus, CRISPR in biotechnology is essentially a tool to recognize and cut DNA at very specific locations for subsequent modification.
Application of CRISPR to crop improvement
It is important to understand that successful application of CRISPR for site-specific gene editing and crop improvement is predicated on a thorough understanding of DNA sequences and protein functionality. Scientists can’t make specific gene edits without first knowing gene sequences and functions. This basic research takes years and is funded by checkoff dollars, federal agency grants and company investments. It is accomplished in the private sector and at universities.
For soybeans, genome editing targets include genes for yield improvement, yield preservation, quality and other traits; such as decreased flower abortion and increased pod set, improved resistance to multiple insects and diseases, herbicide tolerance, improved amino acid composition and custom protein production, optimized oil profiles and accumulation, enhanced nutritional value through decreases in trysin inhibitors and other antinutritional factors like raffinose and stachyose, drought or flooding tolerance, soil salinity or pH tolerance, and improved nutrient uptake and use.
Will crops modified by CRISPR be regulated?
The short answer to this question is “it depends.” Here’s why. First, it will be up to regulatory agencies to determine if and how CRISPR and similar genome editing techniques and edited plants will be reviewed and regulated. In the U.S., regulators have stated that simple mutations created by CRISPR and other genome editing techniques may not be regulated as GMO crops, because natural mutations and those induced by chemicals and radiation previously have not been regulated in crop commercialization. However, the Court of Justice of the European Union (ECJ) recently decided that “organisms obtained by mutagenesis are GMOs and are, in principle, subject to the obligations laid down by the GMO Directive.” If it stands, the ECJ decision will deal a major blow to CRISPR and other genome editing technologies, to the crops developed by these techniques ,and to the companies and universities that have made these investments.
Second, it is important to know that, in most cases, plants must be stably transformed (using the tools and techniques of biotechnology and genetic engineering) to get the guide RNA and the Cas9 nuclease into cells for site-specific gene editing. Those processes make the plants transgenic and there will likely be regulatory review required. For the longer-term, a lot of work is being undertaken to develop systems that will excise the CRISPR sequences from the plant genome after the specific modifications have been made. Other scientists are working with novel DNA delivery systems, like modified plant viruses, to introduce the CRISPR components without incorporating them into the plant’s genome.
Finally, if the CRISPR technique is used to specifically cut the crop plant’s genetic material and insert a new gene that will code for a novel protein, that process and the resulting plants will probably be carefully reviewed and regulated as GMO crops.
Summary
Genome editing technologies like CRISPR are potentially powerful and exciting new biotechnologies that may be used for crop improvement by increasing efficiency, specificity and cost-effectiveness of site-specific gene modification and gene insertion. In soybeans, there have been some biological challenges to adapting these technologies, but those are being addressed. Farmers should keep in mind that this technology, like all others, also has limitations. For example, gene editing depends on a thorough understanding of the plant’s DNA sequences and their protein functions. In addition, plants must be amenable to several genetic engineering processes, the expression of the CRISPR components and the resulting edited genomic sequences. Finally, review and regulation of CRISPR-derived crops will depend on political and legal perspectives, and the processes and products being commercialized.
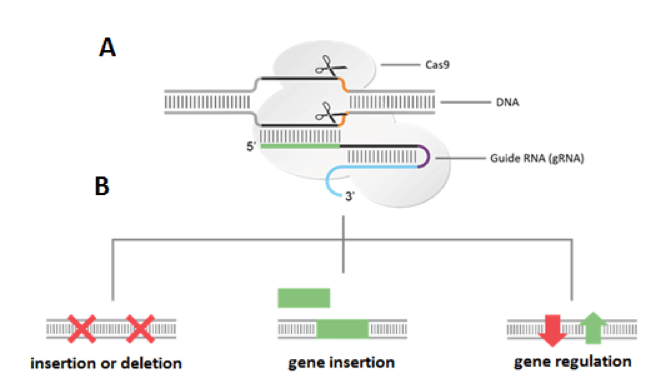
Figure 1B. If random, the mutation usually results in silencing or knocking out (turning off) protein expression from that gene. If specific, the indel mutations may regulate gene expression (gene regulation) for more or less protein. Other specific indel mutations modify genes to produce proteins that have new and better functionality. Finally, if novel genes are provided they may be inserted (gene insertion) into the cut DNA during repair for entirely new beneficial proteins.
Published: Jan 1, 1970
The materials on SRIN were funded with checkoff dollars from United Soybean Board and the North Central Soybean Research Program. To find checkoff funded research related to this research highlight or to see other checkoff research projects, please visit the National Soybean Checkoff Research Database.